Background
Soil salinity has been reported as a major factor to farm land degradation. About 6.7 million hectares are considered salt affected and 72 million hectare are considered sodic in EU[1]. This is twice the area of Germany. The major threats are along coastal areas in the south where intensive use of saline agricultural water is leading to desertification. An EU ComCoast study emphasized the risk in Northern EU countries by sea level rise, that salt intrusion is threatening the coastal areas[2]. In many instances, irrigation causes an increase in soil salinity due to overirrigation of agricultural land, inefficient water use and poor drainage of unsuitable soils. Most known agricultural crop plants are salt-sensitive glycophytes, of which, the growth is severely inhibited when grown under saline conditions. Therefore, these plants cannot be produced economically in salinized soils or with saline water. The United States Department of Agriculture estimates that, worldwide, 10 million hectares of arable land are lost every year to salinity as a result of improper irrigation[3]. 24 % of globally usable land on Earth is degraded at an estimated economic loss of 490 USD billion per year[4]. This is a global and European challenge that needs to be addressed and this challenge will become increasingly demanding in order to meet the expected demand of 50% more food, 40% more energy, and 30% more water by 2030[5] [6] [7].
Halophytes are plants that are salt tolerant and can grow in saline soils and/or be irrigated with seawater. Haophytes e.g. Crithmum maritimum, Portulaca oleracea, Salicornia spp. and Aster tripolium have been consumed by humans for centuries, and are still often gathered from the coastal salt marshes and inland salt pans of Europe. These species are well known for their ability to synthesize high concentration of bioactive secondary metabolites. Hence, this type of vegetables give a high potential for co-production of food and bioactive compounds. A range of cultivation systems for the utilization of halophytes have been developed and commercialized, for the cultivation of gourmet vegetables and purification of saline effluent (e.g. aquaculture effluents).
The secondary metabolites in halophytes include simple and complex sugars, amino acids, quaternary ammonium compounds, polyols and antioxidants (e.g. polyphenols, b-carotene, ascorbic acid and ureides)[8] [9] [10] [11]. These compounds can potentially be utilized in functional food, which is defined as having disease-preventing and/or health-promoting benefits. The modern awareness of a healthier diet promotes additional markets for halophytes with high nutritional potential, evident in the rapidly growing consumption of products from some halophytic plants (e.g. Salicornia and quinoa)[12].
In the AQUACOMBINE project we aim to demonstrate combined aquaculture and halophyte farming (farming of saline tolerant plants) using the principles of circular economy, where waste is recovered and utilised with-in the system to create both internal value and new products, beside avoiding the wastes. Residues are utilised with-in the system to create both internal value and new products. Excess nutrients from the fish production will be used as fertiliser for the halophyte plants and filtered through a microbial water treatment system to enable recirculation of the water back into the aquaculture tanks. All parts of the halophyte biomass will be used for production of multiple products such as food, feed, botanical extracts and pure bioactive compounds, as well as biogas from the final residues to produce energy and a nutrient rich residue to bring essential nutrients (e.g. phosphates) back to farmland. This combined aquaculture, farming, and bioprocessing can help desalinize salt effected areas and can easily be combined with sustainable management of natural areas and/or use of marginal lands to create value and jobs in rual, remote and salt affected and areas. The bioprocessing will create added value to the combined farming and diversify products.
As the advantages of halophyte agriculture is very clear – in terms of utilization of marginal lands – producing health promoting foods – and for bioremediation of saline soils and/or aquaculture effluents there are challenges, which are preventing implementation on a larger scale.
With the proof for greenhouse cultivation and mechanical harvest the use of halophytes as sea vegetables has seen a steady growth in countries like Great Britain, France and the Netherlands. Salicornia europaea L. is used for sea vegetable production. This cultivation requires a high seed density per square meter (2-4g/m2, depending on season). The sowing starts early March till end of April. This offers the opportunity to harvest vegetable from June till end of August. At this stage young fresh tips can be offered. Once the switch in photoperiodicity (mid of September) the plants starts lignifying slowly. From measurements the Salicornia europaea (Sea asparagus/Marsh samphire) crop built up two thirds of its total biomass in the period September – end October/beginning of November. In the first 2 weeks in September the green tips are still very succulent but worthless as vegetable and offers possibilities for fresh plant extracts. Stage 3 can be described as flower initiation and first seed setting. A final stage is seed ripening and die off of the crop. S. bigelovii has a different photoperiodical strategy. From previous experiments, we noticed that S. bigelovii remains vegetative as temperatures remain below 20 degrees.
We must evaluate this feature in terms of a Northern European Salicornia production. Growers could benefit from the valorization of the residue biomass of their cultivated sea vegetables through a longer cultivation period. Intermediate crops will help in keeping the saline soils in shape to cover these soils over a longer period. Hence, in the AQUACOMBINE project, we aim at valorizing the non-food part of the halophyte biomass in terms of non-food applications, and indirect food applications e.g. by aquaculture feed production.
The valorization of the non-food part of the biomass as presented in the AQUA-COMBINE project will be essential in catalyzing new European halophyte businesses.
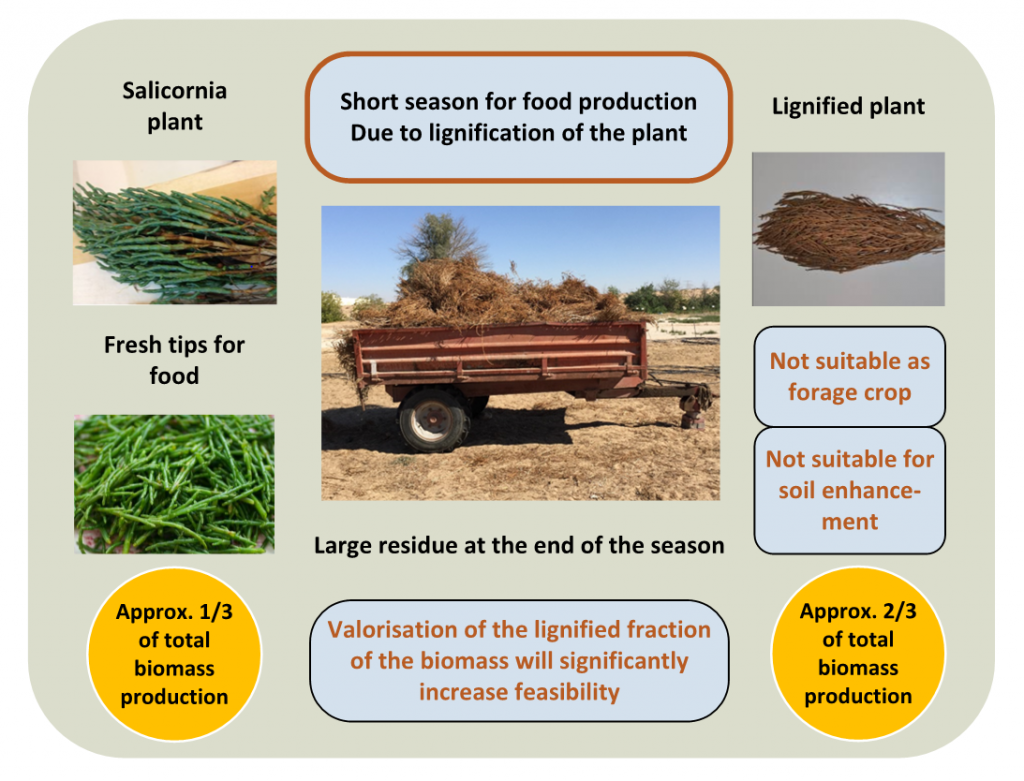
[1] http://www.fao.org/soils-portal/soil-management/management-of-some-problem-soils/salt-affected-soils/more-information-on-salt-affected-soils/en/
[2] Van der Graaf, A. J. and M. J. J. E. Loonen (2006) Onderzoek naar de mogelijkheden voor zilte landbouw in de provincie Groningnen: 57.
[3] Ventura Y. et al. (2015) Annals of Botany 115: 529–540.
[4] www.lambdaconsult.com
[5] Yang, Y.C.E. et al. (2016) J. Water Resour. Plan. Manag. 142 (12), 4016062.
[6] Zhang, X. and Vesselinov, V.V. (2016) Appl. Energy: 183, 77–87.
[7] White, D.J. et al. (2018) Appl. Energy 210: 550–567.
[8] Parvaiz A. and Satyawati S. (2008) Plant, Soil and Environment 54: 89–99.
[9] Ventura Y. and Sagi M.(2013) Environmental and Experimental Botany 92: 144–153.
[10] Stuchlık M. and Zak S. (2002) Biomedical Papers 146: 3–10.
[11] Buhmann A. and Papenbrock J. (2013a). Functional Plant Biology 40: 952–967.
[12] Panta S. et al. (2014) Environmental and Experimental Botany 107: 71–83.
Acknowledgement
This project has received funding from the European Union’s Horizon 2020 research and innovation programme under Grant Agreement No 862834. Any results of this project reflects only this consortium’s view and the European Commission is not responsible for any use that may be made of the information it contains.